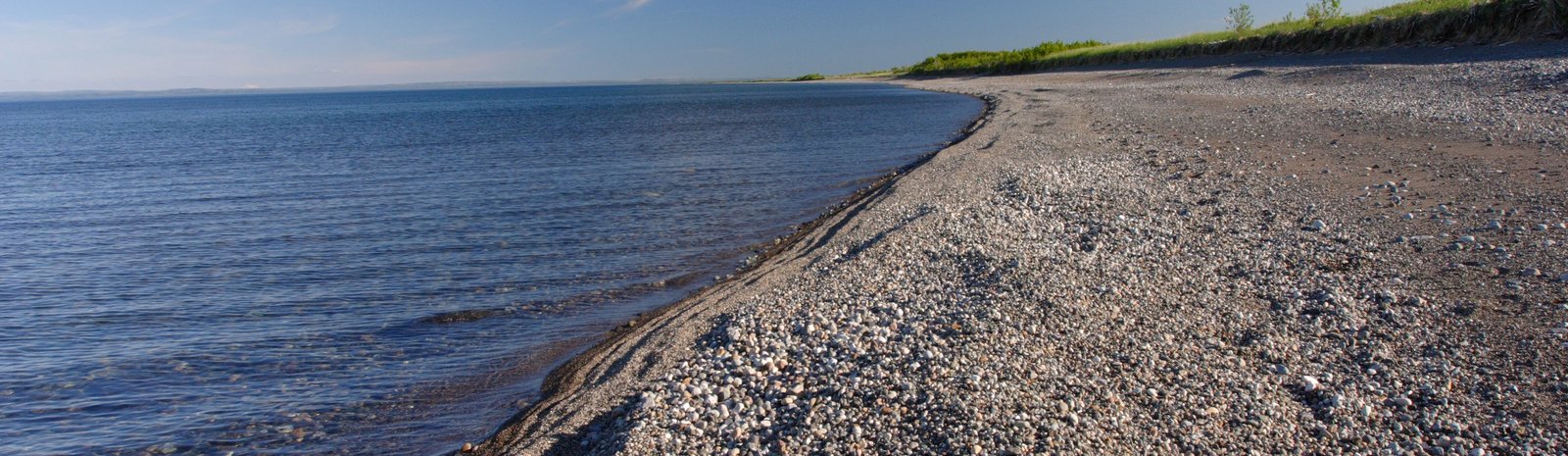
- Abstract
- Introduction
- Terrace survey methods
- A possible active fault near Lake Iliamna
- What are the implications for the Pebble Prospect and local communities if there is an active fault?
- Bibliography
Abstract
Elevated beaches along the shore of Lake Iliamna and Kamishak Bay have the potential to provide constraint on vertical crustal deformation in southwest Alaska (Map 1). Tectonics in this area are little studied, and seismic hazard is of particular interest because of the possible development of Pebble Mine and its associated perpetual tailings storage structures.
Lake Iliamna
Vertical shifts in the ancient beaches surrounding lake Iliamna provide evidence of a potential fault running near the north shore of the lake. We conducted GPS surveys of these beaches, and found that the highest, likely dating to around 26,000 years ago (Stilwell and Kaufman, 1996), drops by about 10 meters over a distance of a few kilometers, though it is nearly flat outside this narrow band. Given that this beach was originally horizontal, the localized change in elevation of the beach must be related to localized deformation of the earth’s crust. This deformation is most likely related to the Lake Clark Fault or a previously undiscovered fault in the area. We plan to conduct further surveys in 2011 to further test this hypothesis.
Kamishak Bay
UNDER CONSTRUCTION: Beaches stranded tens of meters above Kamishak Bay show that this coastline has experienced at least gradual uplift over the last 100 millennia, and there may be evidence for more dramatic recent deformation.
Introduction
Importance
In our fieldwork, we explored possible evidence for geologically recent activity on both the Lake Clark and Bruin Bay faults near Lake Iliamna, as well as searching for unexplored faults in the area. We undertook this fieldwork to address some of the large uncertainties about tectonics in this region, which are particularly relevant to the prospects for industrial development in the area, such as the proposed Pebble Mine.
Assessing activity on a fault
Faults are deeply penetrating fractures in the Earth’s crust. Faulting results when stress builds up and causes the rock to shear. A fault is ‘active’ if that process of building stress is ongoing, and earthquakes are thus likely in the future. This is different from inactive faults, where stress is no longer concentrated and earthquakes no longer occur.
The main way geologists assess whether a fault is active is by attempting to determine whether it has produced earthquakes in the geologically recent past (the past few hundred thousand years). If an earthquake has occurred on a fault recently, then the growing stress that leads to earthquakes is likely still there, and will lead to earthquakes in the future. Given that even very active faults only have an earthquake every few hundred to a few thousand years, and we have only been measuring earthquakes for the last century, it is common that no direct measurements or observations exist of earthquakes on a particular fault. Instead we must rely on evidence that has survived since the most recent earthquake. This geologic evidence becomes part of a detective story - clues that can show an earthquake has occurred.
Many types of evidence can reveal ancient earthquakes. In our fieldwork, we focused primarily on ancient beaches that can provide evidence for vertical motion on a fault. When a beach forms on a lake or the ocean, it is horizontal, and extends only a few meters above the normal water level. So if a beach is found high above the water, or a beach varies in elevation along its length, this may be evidence for tectonic activity.
Regional Tectonics (Map 2)
The tectonic deformation of southern Alaska is driven by collision of the Pacific Plate with the North American Plate. Faults in the area around Lake Iliamna, along with most of Western Alaska, have not been studied in detail. Lake Iliamna straddles the northwest edge of the band of earthquakes and volcanoes that comprises the Pacific ‘Ring of Fire’. It’s the sort of place where active faults are likely, but few earthquake geologists have worked in the field here, and few instruments have been deployed to measure plate motion or earthquakes.
Geologists do know something about tectonic plates in the larger region surrounding Lake Iliamna and the base of the Alaska Peninsula. There is good evidence that most of southcentral Alaska, called the ‘Southern Alaska Block’ (Haeussler, 2008) is a section of the earth’s crust that is moving westward relative to the rest of North America. The Denali Fault, in the Alaska range, is the main fault that this block moves along, but there are other faults, including the Castle Mountain Fault just north of Anchorage, that allow it to deform and move westward. Additionally, there is evidence that the crust beneath the Bering Sea (called the ‘Bering Block’, Mackey et al., 1997), is rotating clockwise relative to North America and eastern Russia. And the Pacific Plate, which extends all the way from the Gulf of Alaska down into the South Pacific, is sliding northwards, beneath the continental crust that forms Alaska.
These three pieces of the earth’s crust surround our field sites. To the east is the Southern Alaska Block, to the northwest is the Bering Block, and to the south is the Pacific Plate. It is unclear whether the Lake Iliamna region is part of the Bering Block or Southern Alaska Block. It may even be that the Bering Block and Southern Alaska Block have no distinct boundary between them (Redfield et al., 2007).
Also, there are known faults in the area that were once active, and which may or may not currently be active. The Lake Clark Fault (Haeussler et al. 2004), an extension of the Castle Mountain Fault, extends southwest from Lake Clark Pass down through Lake Clark. Preliminary work on the northeast end of this fault suggest it hasn’t had an earthquake that broke the surface of the earth since the end of the last ice age (Koehler, personal communication), but there are no published studies establishing whether this fault is active. The Bruin Bay Fault branches from the Castle Mountain and Lake Clark faults near Tyonek, and runs south along the Cook Inlet coast into Katmai National Park. This fault is believed to be inactive because it does not cut an intrusion south of Kamishak Bay (Detterman & Reed, 1980). Neither of these lines of reasoning rule out fault activity below the surface.
Glacial History (Map 3)
The entire region around the study area has been repeatedly inundated by moving ice (Kaufman & Manley, 2004, and Alaska Paleoglacier Atlas). During their advance and retreat these glaciers shaped the landscape by eroding and depositing sediment, scouring out the depths of Lake Iliamna, building moraine ridges, flooding areas along their margins with plains of river gravel, and burying ice that melted to leave kettle lakes. It is against the backdrop of this dramatic reshaping of the landscape that tectonic processes may have had an additional impact.
Glaciers advance and retreat over time, and terminal moraines left at their greatest extent during a given advance provide a widespread indicator of where they were in the past. The most recent ice age followed an interglacial period similar to, but slightly warmer than, the present that extended from about 130,000 to 110,000 years ago (The Sangamon Interglacial). During this time temperatures were about 2° C warmer, and sea levels were about 8 m higher than today (Kopp et al., 2009). The period from about 110,000 years ago to about 12,000 years ago is classified as the Wisconsin glaciation, an “ice age” when glaciers were much more extensive than the present across much of the globe. The greatest extent of ice in the area of this study likely came late in this period, around 26,000 years ago (Stilwell and Kaufman, 1996). At this time ice extended south across Kodiak Island and west well beyond Lake Iliamna. As ice retreated to nearly its current extent sea level rose, reaching approximately its current level about 6000 years ago.
Terrace survey methods
We surveyed transects typically extending from the current beach to uplands above the highest terrace feature using a Trimble GeoXH GPS system. Along Lake Iliamna, this system was supplemented with a Zypher antenna and tripod, while on Kamishak we used the unit on its own with a monopod.
Post-processing of the GPS data using Trimble’s differential correction software correlated with various base stations in the region yielded estimated errors generally under 10 cm both vertically and horizontally. In a few cases where weather or terrain reduced quality vertical error ranged as high as 80 cm. All of these errors are less than the natural variation in geomorphic features.
Our surveys collected points at least at every major break in slope. On Lake Iliamna where beach features are scattered across long smooth slopes we focused on erosional beach-scarps, mapping the breaks in slope at their base and top. On Kamishak better separated terrace features allowed other features to be mapped, including wave-cut platforms, wave-built platforms, and beach ridges.
We identified the areas once inundated by Lake Iliamna on aerial and satellite imagery. Beach features are quite distinct from uplands mottled by glacial kettles or inscribed by braided river channels (See Map 4). On some profiles the only imagery we had available is lower resolution, which adds some uncertainty (see our Detailed Terrace Data Analysis page).
A possible active fault near Lake Iliamna
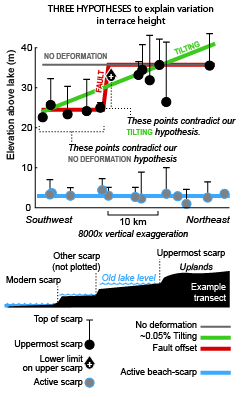
Our survey along Lake Iliamna traces a once-horizontal beach that now varies in elevation. The variation cannot be explained without some deformation of the crust. Crustal deformation in this setting could be caused either by faulting or by tilting following deglaciation. However, the tilting hypothesis is inconsistent with our data, while vertical offset on a fault near Lake Iliamna can explain all our data (Fig. 1). Since the elevated beaches are less than 26,000 years old, offset across them indicates a fault that moved recently enough that it must still be active.
Lake Iliamna is ringed by a series of old beaches, each recording a past when the lake level was higher (Detterman and Reed, 1973). The highest beach likely dates to not long after ice began retreating from the basin now occupied by the lake about 26,000 years ago (Stilwell and Kaufman, 1996). The lake level, then and now, is defined by where water spills out over the remains of glacial moraines at the southwest end of the lake. Over time this water, flowing into the Kvichak River, cut down through the moraine, lowering the lake level and leaving old beaches stranded high above the new water line.
If the crust beneath Lake Iliamna has been distorted up or down during the time since a given beach was formed, that beach could record the change. Each beach was horizontal when it was active, so if a beach varies in elevation along its length, then it must have been deformed.
There are two likely explanations for any deformation revealed by varied elevation of an old beach. Large glaciers like the one that once occupied Lake Iliamna depress the crust downward, and if the beaches formed early enough in the retreat of that ice they would record the rebound of the crust to its current position. This “isostatic rebound” would likely cause relatively uniform tilting of the old beach. Alternately, tectonic deformation from surface or shallow faulting would instead cause vertical variation over a shorter distance, such as by forming a step in the height of a beach.
A preliminary USGS study of the Lake Iliamna elevated beaches (Kaufman & Stilwell, 1995) suggested that there has been no deformation (either isostatic or tectonic). However, these conclusions hinge on a single terrace elevation measurement, and in this case it’s unclear whether the measured surface is actually a beach terrace since no erosional scarp was documented.
In contrast, our more detailed survey demonstrates the opposite. The elevation of the highest beach is consistent along the southwest end of the lake, but steps up by around ten meters further east. This suggests the existence of an east-side up fault near the lakeshore southwest of the outlet of Lower Talarik Creek.
We focused our analysis to the uppermost erosional bank or “scarp” on each profile. Scarps mark the top of beaches during times when the lake level was nearly constant for long periods of time (probably thousands of years). We chose the uppermost scarp because it is easier to correlate. Beach features are quite easy to see on aerial photos (Map 1), and the uppermost feature divides lower terrain marked by beach features from glacially or fluvially modified uplands. However, because scarps erode higher terrain, in they may remove higher scarps or other beach features. So though the base of the upper scarp is in most cases the highest point where we have evidence of a beach, it’s possible that other beach features had higher elevation, but no higher than the top of the observed highest scarp.
Content figure: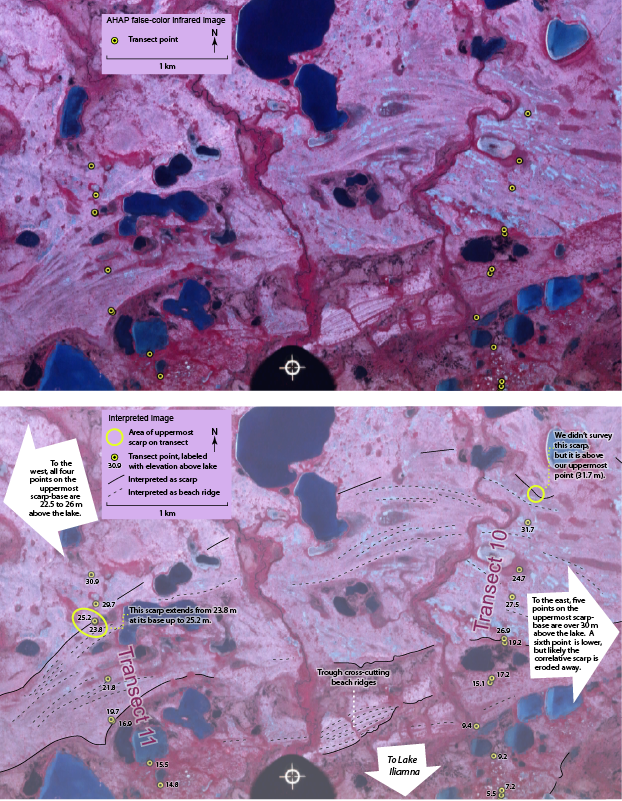
Between transects 10 and 11 (Fig. 2), the height of the uppermost scarp changes by over 6.5 m across only a few kilometers of lakeshore, which we interpret as a vertical shift along a fault running between the two transects. Transect 10 documents several scarps, but the highest point on that transect is still below the uppermost scarp. That point is 31.7 m above modern lake level. If the highest scarp had been measured, the difference in height from the western half of the old lakeshore would be even more extreme. Just a few kilometers southwest of Transect 10 we surveyed Transect 11 to the highest beach and beyond it. The base of the highest scarp is at 23.8 m, and even its top is only 25.2 m above the lake. Conservatively, the uppermost beach must have changed elevation over a few kilometers by over 6.5 m. Changes on the order of a meter due to differences in the beach are not uncommon, as can be seen in our surveys of the modern beach scarp, but a minimum of 6.5 meters is far beyond any variation we observed or expect.
Looking northeast and southwest of these transects, other transects add weight to this pattern. To the east, in all but one of our observations the uppermost scarp base (a minimum height for the upper beach) is over 30 m. The only exception, on transect 5, is a scarp that is exceptionally tall. In Landsat satellite imagery (via Google Earth) higher beaches just to the east can be seen to terminate at the top of this scarp, showing that it eroded these higher beaches at this transect. To the southwest our transects all have upper scarp-bases that are 22.5 to 26 m above the lake. However, it is possible in several cases that the upper scarp coincidentally eroded an even higher scarp more in line with points northeast of Transect 11.
Conclusions
To explain the data from our fifteen elevation transects along Lake Iliamna, we considered the possibilities that the shore of Lake Iliamna was either stable, tilted, or deformed more complexly by faulting. The only hypothesis of these that could reasonably explain our data, and particularly our transects 10 and 11, was faulting. So we tentatively conclude that there is an active, east-side-up fault near the shore of lake Iliamna.
To confirm or disprove our conclusion that terraces along Lake Iliamna are deformed by an active fault, we will conduct an additional survey. The results presented here were collected with no knowledge of what points would be most crucial to delineating deformation. A return survey could focus on the area where there appears to be crustal deformation. We plan to survey additional points on prominent scarps, and trace the crests of beach ridges. Also we’ll survey prominent features to better match detailed aerial photos with our survey. Our goal is to complete this survey by summer 2011.
For a detailed analysis of our data and data from Kaufman and Stilwell, 1995, see our Detailed Terrace Analysis page.
2011 Survey plans
We will be conducting additional GPS surveys to test and clarify our results. Map 6 shows traces of possible features we might survey. This work will fill in detail right in the area of the possible fault, providing a stronger test of our hypothesis, and providing further constraint on the orientation and location of the fault.
This survey would take several approaches to testing and adding detail to our evidence for a fault. Blue marks survey of the upper scarp, black marks other areas of the elevated beaches, yellow marks beach and braid plains that may show evidence of tilting, and green marks points collected to improve rectification of aerial imagery. The actual survey would target a subset of these possible field sites.
What are the implications for the Pebble Prospect and local communities if there is an active fault?
This possible fault lies near a large copper and gold mining prospect called the Pebble Prospect. Initially, the Pebble Partnership assumed the closest possibly active fault to their prospect was the Lake Clark Fault, and they suggest that it lies no closer than 18 miles from the proposed mine. However, if the apparent tectonic deformation we document is in fact related to motion on the Lake Clark Fault, then the fault runs only 5 miles from the proposed pit, and crosses through an area that would likely be used for tailings impoundment. Shaking from earthquakes increases near the source fault, so this suggests that the Lake Clark Fault is a potentially much greater concern for the mine than they originally assumed. Worse yet, if the fault actually crosses under a tailings facility, then it could directly offset tailings dams or other infrastructure, destroying their structural integrity.
This is particularly critical for the design of the tailings impoundment facility. The preliminary design includes multiple dams up to 700 feet tall intended to contain the metal sulfide tailings generated by the mine. To prevent the release of billions of tons of acid and metal generating material into downstream waters, these dams will need to remain intact in perpetuity, both during mine operation and following mine closure. To address the greater hazard posed by a fault nearer than previously thought, dams and other structures at the facility would need to be built to withstand a higher intensity of shaking than initially estimated.
It’s also possible that we are documenting evidence of a fault that is not the Lake Clark Fault, and which may or may not be related to motion on that fault. If this is the case, our survey directly documents deformation 22 miles from the mine site, and closer than that to possible tailings sites.
For communities near the fault line, particularly Nondalton and Igiugig (both within a few miles of the likely Lake Clark Fault trace), the fault could present a major hazard. Though it may be generations before the next earthquake occurs here, it could also happen any time. Some suggestions for preparing for earthquakes in your area are provided by the Alaska Earthquake Information Center.
Bibliography
- G. Carver, J. Sauber, W. Lettis, R. Witter, B. Whitney, 2008: Active Faults on Northeastern Kodiak Island, Alaska; Geophysical monograph vol. 179, p. 167-184.
- R. L. Detterman, B. L. Reed, 1980: Stratigraphy, Structure, and Economic Geology of the Iliamna Quadrangle, Alaska; Geological Survey Bulletin 1368-B.
- R. L. Detterman, B. L. Reed, 1973: Surficial Deposits of the Iliamna Quadrangle, Alaska; Geological Survey Bulletin 1368-A
- P. J. Haeussler, 2008: An Overview of the Neotectonics of Interior Alaska: Far-Field Deformation From the Yakutat Microplate Collision; Geophysical monograph vol. 179, p. 83-108.
- P. J. Haeussler, R. W. Saltus, 2004: 26 km of Offset on the Lake Clark Fault Since Late Eocene Time; U.S. Geological Survey Professional Paper 1709–A.
- D. S. Kaufman, W. F. Manley, 2004: Maximum and Late Wisconsin glacier extents across Alaska, U.S.A.; from Quaternary Glaciations—Extent and Chronology, Part II: North America; eds. J. Ehlers, and P. L. Gibbard, Developments in Quaternary Science vol. 2 p. 9-27. [see also: Alaska Paleoglacier Atlas]
- D. S. Kaufman, K. B. Stilwell, 1995: Preliminary Evaluation of Emergent Postglacial Shorelines, Naknek and Iliamna Lakes, Southwestern Alaska; from Geologic Studies in Alaska by the U.S. Geological Survey, 1995; eds. J. A. Dumoulin and J. E. Gray, USGS Professional Paper 1574, p. 73-81.
- R. E. Kopp, J. F. Simons, J. X. Mitrovica, A. C. Maloof, M. Oppenheimer, 2009: Probabilistic assessment of sea level during the last interglacial stage; Nature vol. 462 no. 17, p. 863-867.
- K. G. Mackey, K. Fujita, L. V. Gunbina, V. N. Kovalev, V. S. Imaev, B. M. Koz’min, L. P. Imaeva, 1997: Seismicity of the Bering Strait region: Evidence for a Bering block; Geology vol. 25, no. 11, p. 979-982.
- T. F. Redfield, D. W. Scholl, P. G. Fitzgerald, M. E. Beck Jr., 2007: Escape tectonics and the extrusion of Alaska: Past, present, and future; Geology vol. 35, no. 11, p. 1039-1042.
- K. B. Stilwell, D. S. Kaufman, 1996: Late Wisconsin History of the Northern Alaska Peninsula, Southwestern Alaska, U.S.A.; Arctic and Alpine Research vol. 28 #4, p. 475-487.
Further Reading
Created: July 25, 2019